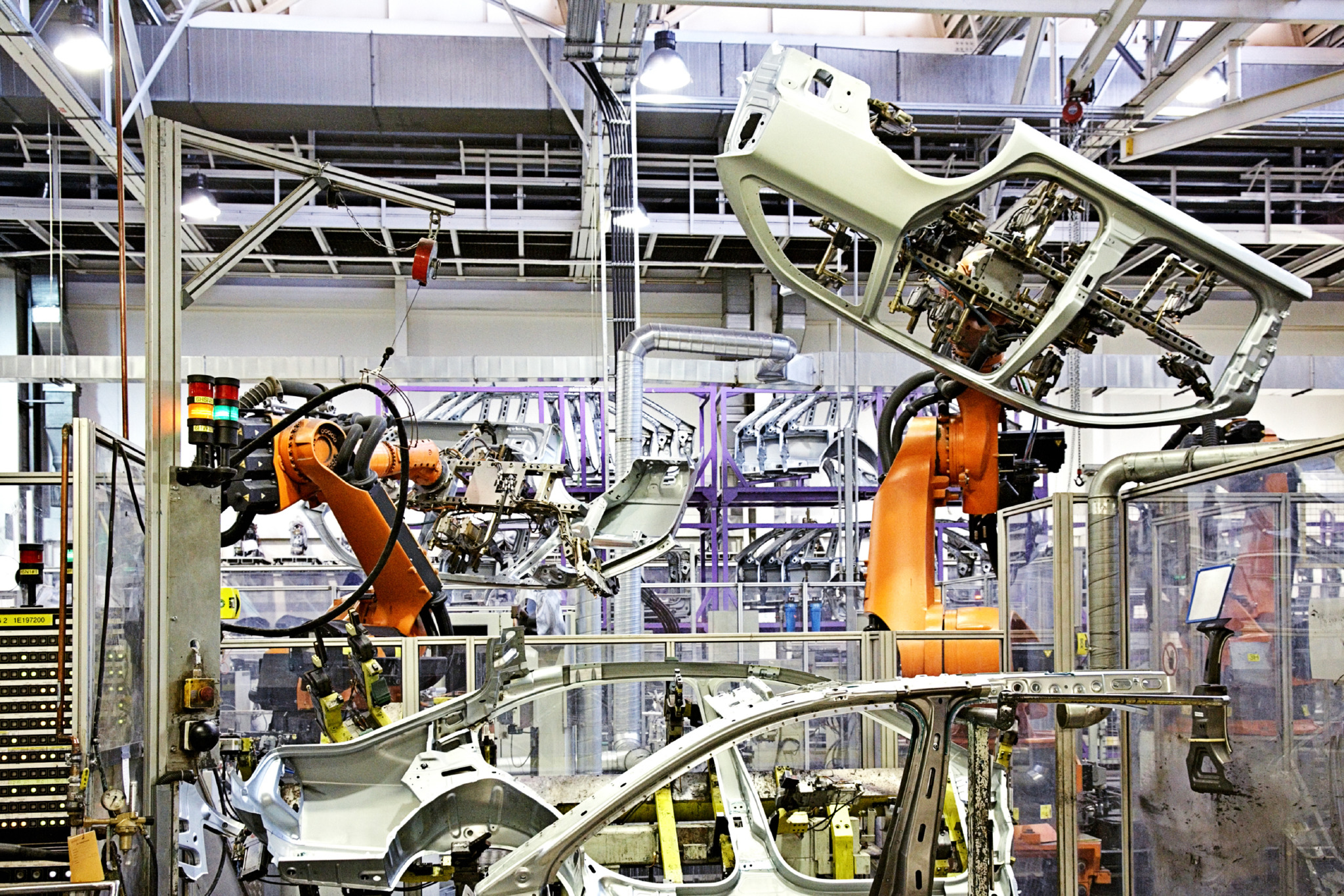
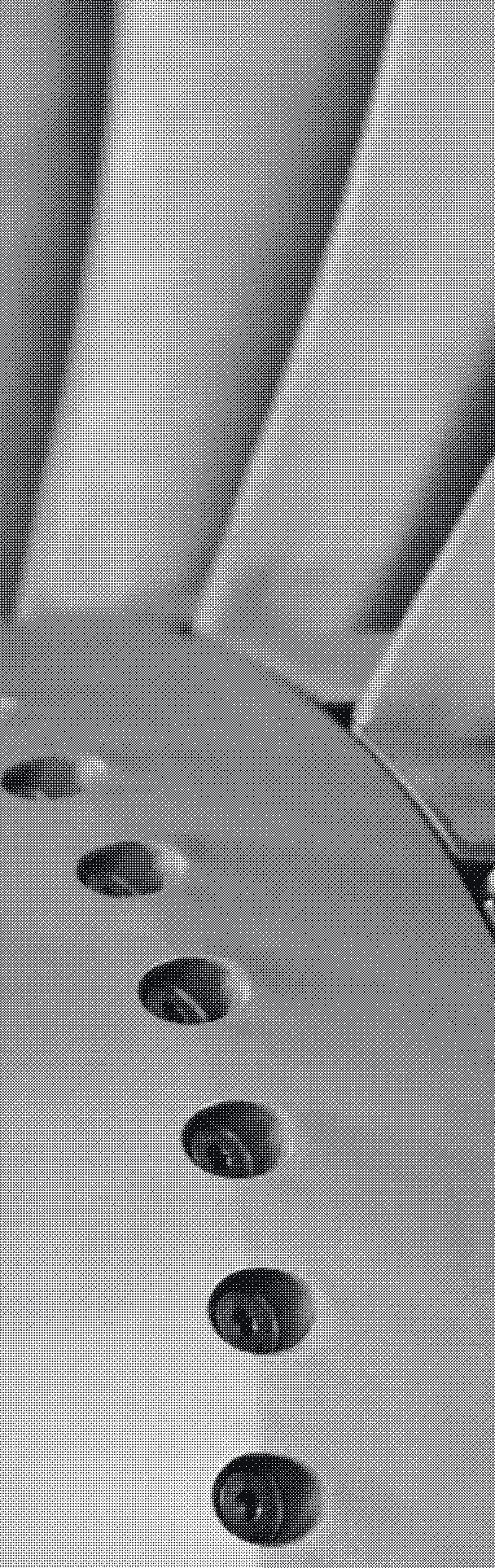
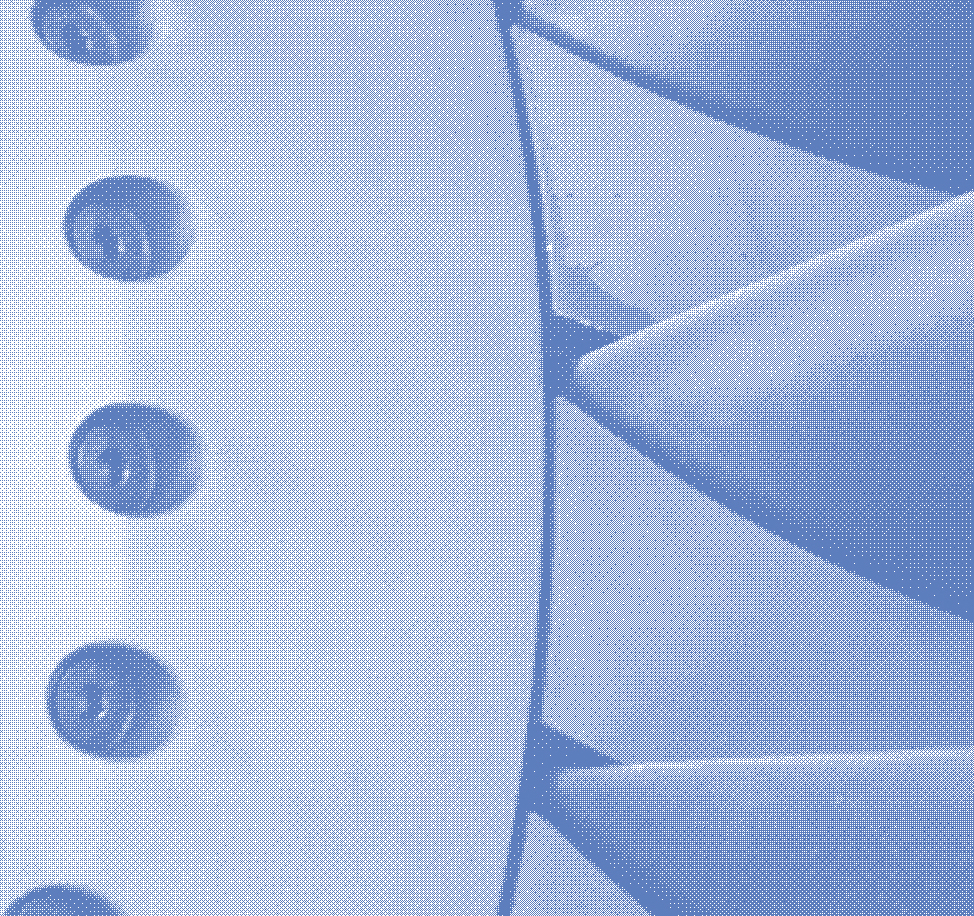
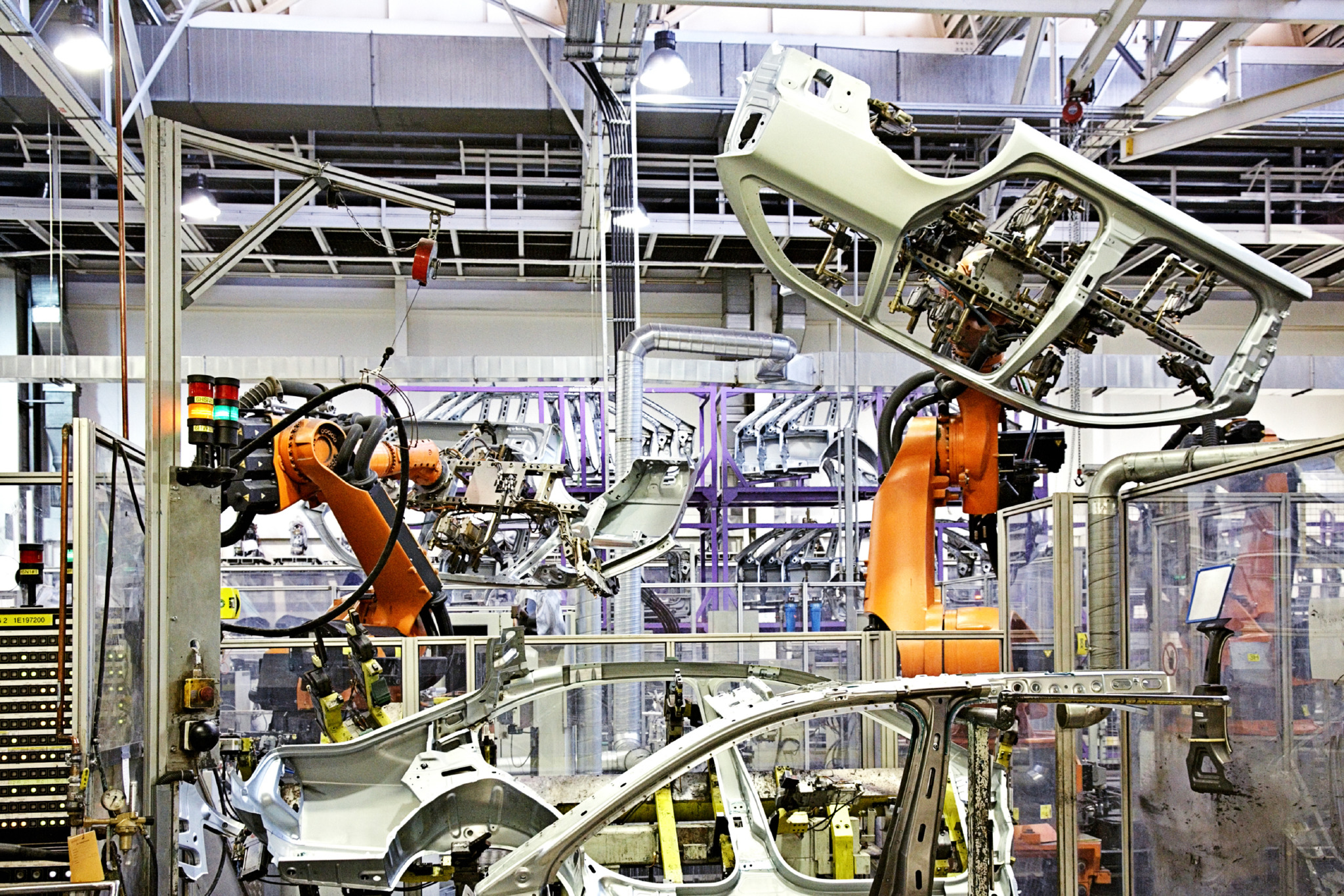
Research Projects
Titanium and Magnesium
Advanced characterisation of deformation micromechanics and failure initiation in Ti alloys
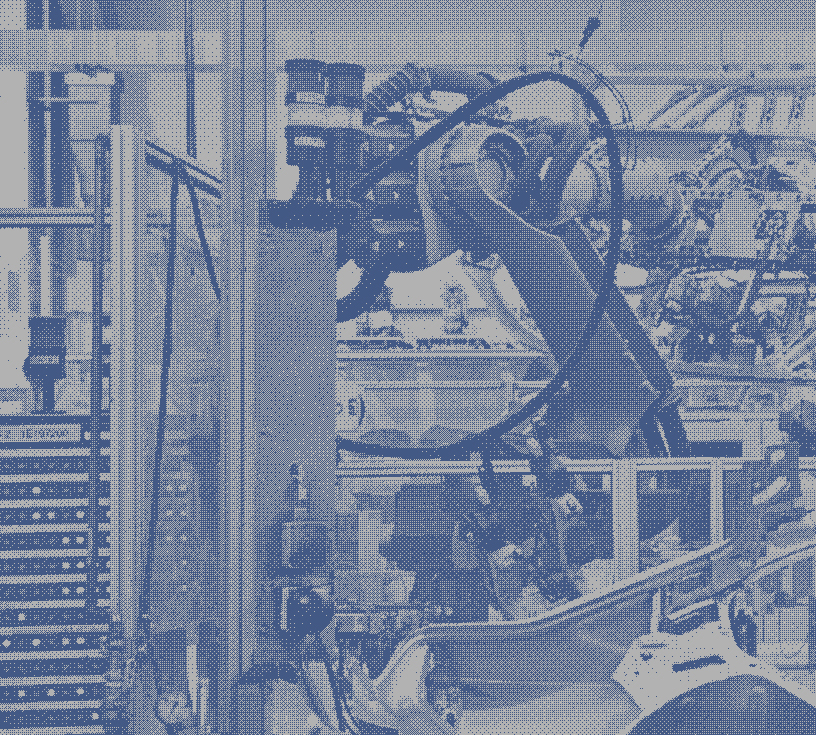
Failure initiation during monotonic and cyclic loading of polycrystalline metallic materials alloys involves irreversible deformation with increasing applied stress, manifested in localised slip bands at the scale of individual grains. In Ti the allotropic phase transformations lead to a complex interplay of morphological and orientation relationships between the high-temperature bcc phase and the low-temperature hcp phase and a great variety of microstructures, having impacts on the monotonic and fatigue properties. To develop more accurate and realistic predictive models for the deformation and failure behaviour of Ti alloys, it is necessary to quantify the plastic strain heterogeneities at the microscopic scale and subsequently relate these values to the critical microstructures. Recent advances in imaging techniques in conjunction with digital image correlation have opened new ways in which we can now quantify strain localisation for the first time, which leads to material failure. A feasibility study has demonstrated that we can even monitor microplasticity in the high cycle fatigue regime, i.e. initial shear strain levels well below 1% with a spatial resolution better than 100 nm. We now want to exploit this new methodology and monitor strain localisation in hot-deformed titanium alloys typically used in the aerospace industry. A particular challenge within the project will be the correlative microscopy aspect that we will bring together 2D strain maps, crystallographic information and local grain rotation recorded in polycrystalline titanium alloys to calculate stress intensities at potential failure sites like grain boundaries and correlate strain localisation with crack initiation. In addition, considering the influence of realistic 3D microstructures, i.e. microtextured regions in mm-scale, on deformation behaviour is a significant challenge. The new Royce-funded plasma-FIB exhibits material ablation rates several orders of magnitude faster than Ga+ FIB and even Xe+ plasma FIB enabling the volumes of material machined into the mm-scale. This project will develop a synergistic approach of combining a range of multidimensional characterisation techniques, micro-test and analysis to establish a new mechanistic understanding of Ti alloys and, more importantly, apply a similar approach to other polycrystalline materials.